Thermal conductivity
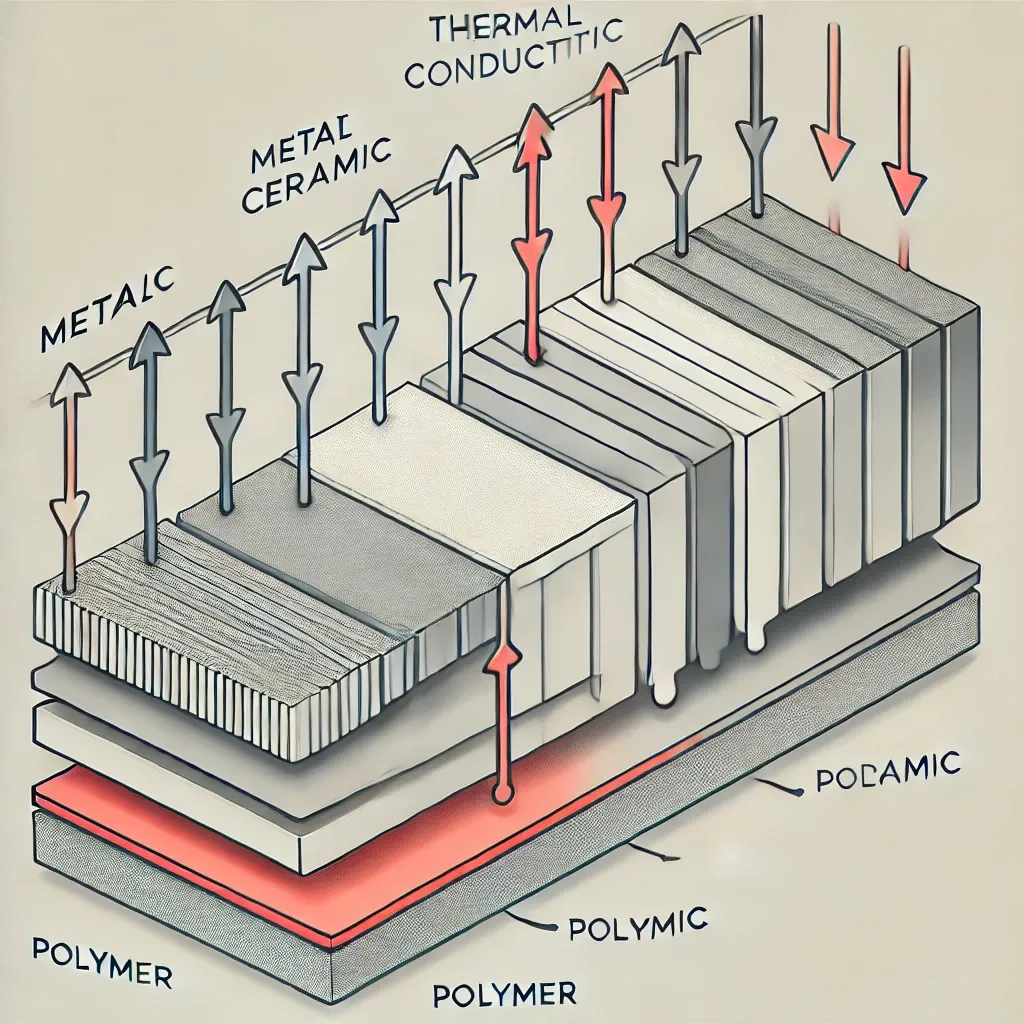
Thermal conductivity (λ) is a material property that quantifies its ability to conduct thermal energy. It is a crucial parameter in thermophysical sciences, determining the rate at which heat is transferred across a material under a temperature gradient. Metals, such as copper and aluminum, exhibit high thermal conductivity due to the efficient movement of free electrons, whereas materials like wood or polymers exhibit low thermal conductivity, making them effective insulators. Understanding thermal conductivity is essential for optimizing thermal management in systems ranging from microelectronics to large-scale structural engineering.
Defining Thermal Conductivity
Thermal conductivity is governed by Fourier's Law of Heat Conduction, which posits that the rate of heat transfer per unit area (ḥ) is proportional to the negative temperature gradient (∇T) and the material's thermal conductivity (λ). Mathematically, this relationship is expressed as:
ḥ = -λ ∇T
In essence, the thermal conductivity of a material determines how effectively it can transfer thermal energy under a given temperature differential. Materials can be broadly classified as conductors or insulators based on the magnitude of their thermal conductivity, influencing their suitability for various engineering applications.
Factors Affecting Thermal Conductivity
- Temperature Dependence: The thermal conductivity of metals generally decreases with increasing temperature due to enhanced lattice scattering of free electrons. Conversely, for non-metallic materials, conductivity is primarily facilitated by phonons (quantized lattice vibrations), and thermal conductivity can exhibit complex temperature dependence. At intermediate temperatures, non-metals may demonstrate relatively stable conductivity, while at extremely low temperatures, phonon scattering decreases, leading to reduced thermal conductivity.
- Phase Transitions: The thermal conductivity of a material can change significantly during phase transitions. For instance, the thermal conductivity of water in its liquid state is considerably higher compared to ice due to the more effective energy transfer between freely moving molecules as opposed to a rigid crystalline structure.
- Crystalline Structure and Anisotropy: The internal structure of a material significantly influences its thermal properties. Anisotropic materials, such as graphite or sapphire, exhibit direction-dependent thermal conductivity, which is a crucial consideration in applications requiring controlled heat dissipation. Impurities and defects within the lattice structure can also scatter phonons, thereby diminishing overall thermal conductivity.
Comparative Analysis of Thermal Conductivities
Thermal conductivity varies dramatically across materials, reflecting differences in atomic bonding, crystal structure, and electronic properties:
- Graphene: Exhibits exceptional thermal conductivity, reaching up to 5300 W/m·K. This makes it a prime candidate for advanced cooling solutions in high-performance electronics.
- Diamond: Known for its thermal conductivity of approximately 2200 W/m·K, diamond is utilized in specialized applications requiring rapid heat dissipation, such as semiconductor heat sinks.
- Common Materials: Everyday materials demonstrate significantly lower thermal conductivities—water (0.6 W/m·K), wood (0.1-0.2 W/m·K), and glass (1 W/m·K) are examples where lower thermal conductivities serve purposes like thermal insulation and energy conservation.
Applications of Thermal Conductivity
- Electronics and Microelectronics: Efficient thermal management is crucial in electronic devices to prevent overheating. High-conductivity materials, such as copper, aluminum, and graphene, are extensively used to dissipate heat from processors and integrated circuits, ensuring reliability and longevity.
- Building and Construction: The selection of materials with appropriate thermal conductivities is pivotal in the construction industry to optimize energy efficiency. Insulating materials like fiberglass and expanded polystyrene are used to minimize heat loss, maintaining indoor thermal comfort with minimal energy expenditure.
- Energy Systems: In power generation and thermal energy storage systems, materials with high thermal conductivity are essential to facilitate efficient heat exchange. Heat exchangers, typically made from metals with high thermal conductivities, enable effective energy transfer between fluids, enhancing system efficiency.
Conclusion
Thermal conductivity is a key determinant of a material's capability to conduct heat, significantly affecting the design and functionality of technologies in diverse fields, including electronics, construction, and energy systems. A profound understanding of thermal transport phenomena enables engineers and scientists to make informed material selections that enhance the performance, safety, and energy efficiency of thermal systems.
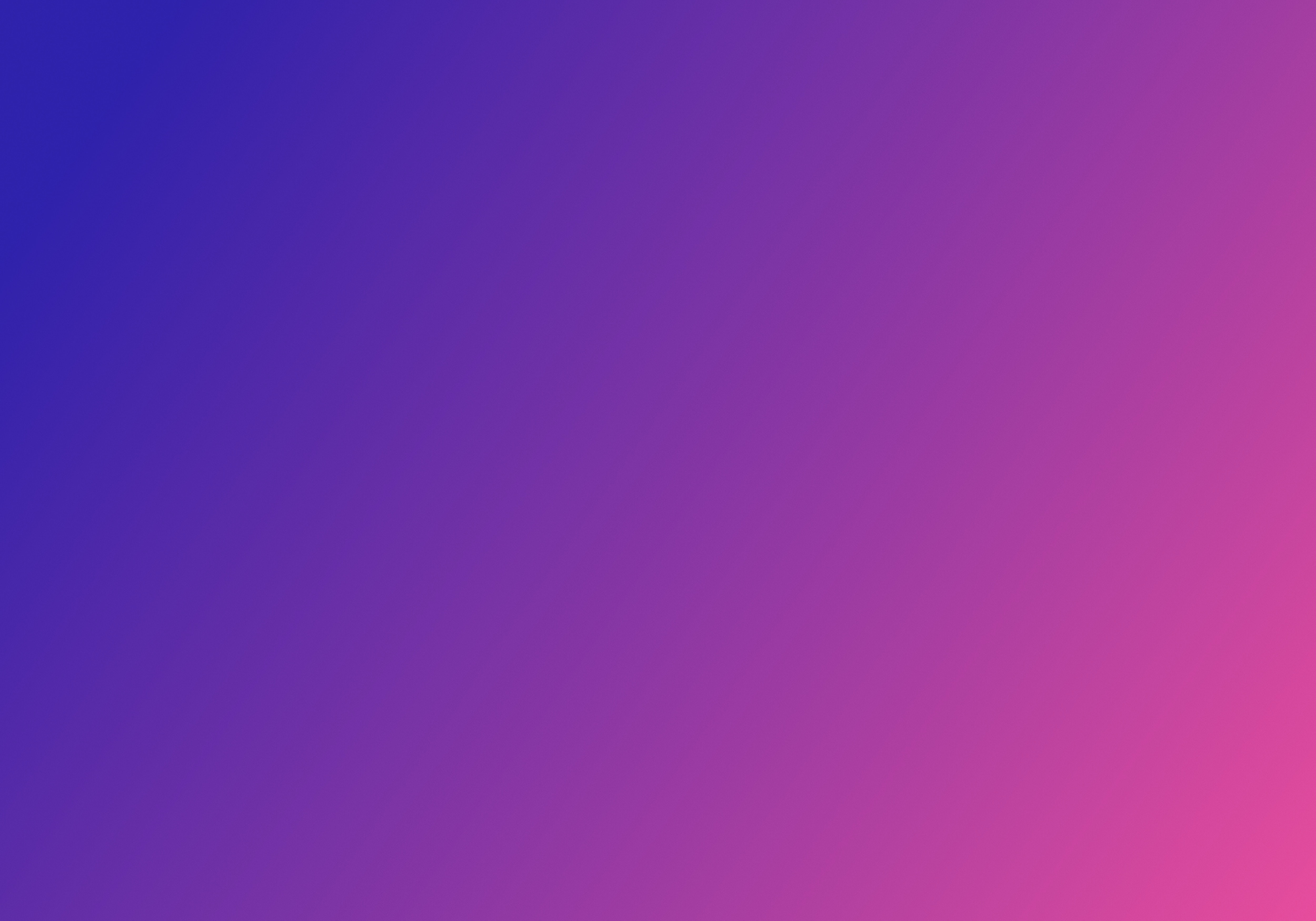
Checkout top 10 thermal conductivity of materials